COPYRIGHT 1999
FUSION
POWER
BASICS OF
FUSION:
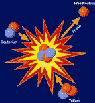
|
THE
PRINCIPLE BEHIND FUSION
CLICK ON IMAGE
FOR LARGER VIEW. (SIZE ?)
|
For elements to fuse together, they
must come so close together that they feel the strong
nuclear force. As they naturally repel one another, they
need a large amount of kinetic energy to overcome their
mutual repulsion. They get this kinetic energy if their
temperature reaches a certain amount. Along with
temperature, density and confinement are important
factors to cause fusion. These are obtained in a plasma.
A plasma is a state of matter where the electrons are
stripped from their host nuclei. In the Sun, fusion takes
place at about 10-15 million degrees centigrade. However
here on Earth the most suitable fusion reaction is that
of Deuterium (D) and Tritium (T). Both of these are
isotopes of Hydrogen. Deuterium occurs once in every 6700
Hydrogen atoms and so can be separated from all kinds of
water. Tritium on the other hand is rare because it is
naturally radioactive and so decays quickly. Tritium can
be manufactured by bombarding Lithium with neutrons. This
can then be incorporated into a nuclear reactor as a
blanket around the containment vessel. The reactor would
then breed its own fuel. The Deuterium-Tritium reactions
occur around 100 million degrees centigrade.
The reason that Deuterium and
Tritium are used in these reaction is the fact that each
ion only has one positive charge and so the mutual
repulsion is minimal. In a fusion reactor Deuterium and
Tritium produce an alpha particle and a neutron with a
combined energy of 17.6 million electron Volts. Four
fifths of this is the kinetic energy of the neutrons.
Because they are neutral, they can pass through the
plasma with virtually no interaction. When they reach the
chamber walls, they slow down and give their energy in
the form of heat to the moderator (Lithium) built into
the walls. This then produces more Tritium and the energy
is taken out by a heat reservoir which by using a
conventional steam turbine, produces electricity. The
remaining 20% of the fusion energy is taken away by alpha
particles. As these are charged, they dont escape
very easily and remain within the chamber giving their
heat to the fuel. The absorption of this energy by the
fuel propagates more thermonuclear burning and if the
density and radius if the fuel is large enough , a
condition called bootstrap heating is
reached. This is the point where the fuel is said to have
ignited.
TWO TYPES OF
REACTOR:
There are two types of fusion
reactor being developed at the moment: the magnetic
confinement reactor and the inertial fusion reactor.
There are several types of magnetic reactors being used
around the world but the two main ones are the Tokamak
and the Stellarator. Both these use magnetic fields to
confine the plasma inside a chamber. If it didnt
the chamber walls would melt under the immense heat of
the plasma. The other type, the inertial fusion reactor
fires laser beams at fuel pellets to cause the nuclear
fusion process to begin. Both of these systems have to
abide by what is called the Lawson condition. This is an
equation that defines the density and confinement time
that a fuel pellet must have for fusion to occur. In
inertial confinement experiments the confinement time is
of the order of a billionth of a second and density much
higher than Lead. For magnetic confinement experiments
the confinement time is of the order of seconds with a
density much less than air.
BASICS OF MAGNETIC
CONFINEMENT:
There are two main methods for
plasma containment, the Torus and the Mirror.
THE
TORUS:

|
THE
TORUS
CLICK ON IMAGE
FOR LARGER VIEW. (SIZE ?)
|
The magnetic lines of force in a
Torus are closed, that is the field lines go around the
Torus and meet back where they started. Every charged
particle is confined to one of the lines. Actually these
particles gyrate around the lines, but can move freely
along it. As the particle trajectory meets itself, it is
trapped. In a Tokamak, two magnetic fields are set up, a
toroidal and a poloidal. The toroidal field magnet
circles the Torus, there are about 16 of them. The
poloidal field magnets are looped above and below the
Torus. The plasma current is induced by a set of Ohmic
heating coils that lie in the centre of the Torus. As the
flux is increasing, the current flows. A Tokamak can
confine a plasma at a density of about 1014 fuel
particles per cubic centimetre. Some heating is produced
by the electrical resistance to the current flowing
through the plasma, but this is insufficient to cause
fusion.
Two methods of heating are used,
radio frequency and neutral particle injection. The radio
frequency heating method uses coaxial cables or
waveguides to set up standing waves with the particles
within the plasma. These standing waves oscillate enough
to heat the particles to the right temperature for
fusion. The other method uses Hydrogen or Deuterium ions
that are accelerated into the plasma. As these particles
exchange energy with the particles in the plasma, they
heat up. This method was used at the TFTR (Tokamak Test
Fusion Reactor) in Princeton to produce 10 million Watts
of power for half a second.
THE
MIRROR:

|
THE
MIRROR
CLICK ON IMAGE
FOR LARGER VIEW. (SIZE ?)
|
This method of confinement acts as
a bottleneck using magnetic field lines. As
the plasma is confined to the field it is essentially
trapped within it. Although some particles can escape at
either end, it is possible to introduce another mirror to
plug this gap. The field lines have peaks at either end
and so a particle conserving energy and angular momentum
converts its energy and motion parallel to the field into
energy and motion perpendicular to the field. As the
plasma is confined, it can be heated by using radio
frequency heaters or ion injectors.
JET:
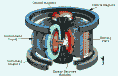
|
THE
JOINT EUROPEAN TORUS
CLICK ON IMAGE
FOR LARGER VIEW. (SIZE ?)
|
The JET (Joint European Torus)
program is a Europe wide program of magnetic confinement
fusion. Like the MEDUSA project, it uses what is called a
Tokamak which is Russian for
TOroid-KAmera-MAgnit-Katushka which means toroidal
chamber and magnet coil. JET is a large Torus shaped
chamber based at Culham near Oxford.
Its primary objectives are to
study:-
- Plasma heating up to
thermonuclear temperatures.
- Plasma behaviour at these
temperatures.
- The interactions of the plasma
with the chamber walls.
- The refuelling of the
plasma.
- Exhaust waste and safety of the
reactor.
- Control of any impurities
within the plasma.
- Deuterium-Tritium
experiments.
- Development for the
International Thermonuclear Experiment Reactor
(ITER)
The achievements so far
are:-
- To construct the reactor on
time and close to its initial budget.
- A record plasma current of 7.1
million amps.
- To reach the sort of plasmas
needed in a fusion reactor.
- The temperature, density and
confinement for a reactor. These were only achieved in
separate experiments.
- Using Deuterium plasma,
conditions for break even (fusion power
produced to equal plasma losses).
In addition to these achievements,
JET produced 1.7 million Watts of fusion power for 2
seconds in 1991. This was done using a 11% mix of Tritium
injected into the Deuterium plasma.
The parameters in these experiments
were:-
- 3.1 million Amps of plasma
current.
- 2.8 Tesla field.
- 200 million degrees centigrade
heating.
- 2 to 4´1019m-13 plasma
density.
This produced 6´1017 neutrons
per second.
In 1997, a mix ratio of 50/50
Deuterium-Tritium was used in three months of
experiments.
These experiments
achieved:-
- 16 million Watts of fusion
power.
- A ratio of fusion power
produced to input power of 65%.
- There is a 25% reduction in the
power needed to maintain the confined
plasma.
- The process needed to breed
Tritium for future reactors.
MEDUSA:
This is classed as a low aspect
ratio Tokamak. Its major radius ranges from 10 to 24
centimetres and its minor radius from 6 to 9 centimetres.
This gives it an aspect ratio of about 1.5. Other low
aspect ratio Tokamaks have a ratio of about 3. Low aspect
ratio Tokamaks have many advantages over conventional
Tokamaks in that they produce a plasma that is more
elongated, have a higher plasma current density, have a
natural divertor for waste removal and are more robust.
One main problem with these Tokamaks is the size of the
central core. As it is small, only the Ohmic heating
solenoid and the Toroidal field coils are passed through
as they are needed. Although MEDUSA is only an economic
tester for larger projects, it has had quite good
success.
Some of these are:-
- Toroidal field strength of 0.45
Tesla
- Plasma current of 40
kiloAmps
Further research is in progress to
Determine such aspects as plasma stability, high plasma
current and sustainment.
BASICS OF INERTIAL
FUSION:
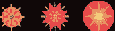
|
THE
PRINCIPLE OF INERTIAL FUSION
CLICK ON IMAGE
FOR LARGER VIEW. (SIZE ?)
|
This process uses lasers to start
the fusion reaction. Firstly a pulse of short wavelength
laser light is split into several beams of equal
intensity. These beams are amplified and by using mirrors
and lenses are brought back together at a focal point.
Placed at this point is a pea sized pellet of Deuterium
and Tritium. As the pellet is illuminated from all
directions with high intensity light, the outer coating
of plastic or glass is very quickly ionised. This layer
of plasma shoots outwards at a speed of about 1000
kilometres a second. Because of Newtons Third Law,
an equal but opposite force contracts the inner core to
more than 50 times it original size. This heats the
pellet and increases its density enough for it to
fuse.
The two types of laser that were
used in fusion research were the Neodymium-doped glass
and the Carbon Dioxide gas lasers. Both of these are
Infra Red lasers and have a wavelength of 1 and 10
microns respectively. These lasers and others of longer
wavelength heat the core of the fuel pellet before the
plasma has chance to expand. This happens because
suprathermal electrons travel through the outer coating
into the core. This premature heating decreases the
density in the core and lessens the chance of fusion to
occur. This was avoided by developing lasers of shorter
wavelength than Infra Red. This was achieved by growing
perfect crystals and cutting them at precise angles to
the orientation of the crystal lattice. The crystals when
placed in front of the incident beam produce frequencies
2 or 3 times the input frequency. If a number of these
are used, the wavelength of the laser is shortened by the
same factor.
An experiment in France used two
crystals of Potassium Dihydrogen Phosphate (KDP) with a
laser of 1 micron to produce a wavelength with a
reduction of a factor of four. When directed at a pellet
it was found that it absorbed nearly all of the incident
energy and no suprathermal electrons were detected. This
was a massive breakthrough for inertial fusion energy
especially with the fact that the most recent laser using
Krypton-Fluoride can produce a beam of only 0.25 microns.
The only downfall of using the frequency conversion
crystals is that it takes up to a year to grow a crystal
of 30 centimetres in diameter. Also in theory a large
number of crystals could be used to keep decreasing the
wavelength of the laser beam, but crystals that transmit
light shorter than 0.2 microns are not yet
available.
OMEGA AND
NOVA:
The OMEGA program is much the same
as the NOVA program. It consists of a number of laser
devises which direct their beams at the fuel pellet.
However in the NOVA experiments it uses what is called
the Hohlraum effect to create great amounts of fusion
energy. This process uses a Deuterium-Tritium pellet that
is coated in a high atomic number material like Lead or
Gold. When the lasers are incident on the pellet the
outer layer converts the beam into X-rays which compress
the fuel capsule.
The OMEGA apparatus can deliver
more than 40,000 Joules of energy onto a target of less
than 1 mm in size in less than 700 Pico seconds. This
produces around 60 Tera Watts.
NIF (NATIONAL IGNITION
FACILITY):
When fully developed this will
house the most powerful Neodymium glass lasers in the
world. It will be 50 times more powerful than the NOVA
laser system. The lasers are split into 192 independent
beamlets which are amplified to heat the pellet up to 50
million degrees centigrade. This will be sufficient to
not only cause ignition, but will also produce a
sustainable reaction.
NIF comprises of two different
target mechanisms:-
DIRECT-DRIVE:- Where the
laser beams are targeted at the Deuterium-Tritium
capsule.
INDIRECT-DRIVE:- Used in the
NOVA system the pellet will be coated in a heavy metal
that produces X-rays and implode the fuel
pellet.
NIF will be able to produce better
results in higher density physics than have been achieved
anywhere else. This will allow scientists to study the
physics of stellar objects such as supernova as well as
developing systems for future nuclear fusion
reactors.
FUTURE
PROPOSALS:
The JET Tokamak at Culham is being
used as a research model for a future reactor called ITER
(International Thermonuclear Experimental Reactor) which
is research reactor being developed for a possible future
nuclear fusion device.
Also at Culham is the START
Tokamak. This is a spherical Tokamak that has a toroidal
field supplied by a central column. It is about 2 meters
by 2 meters in diameter and has an aspect ratio of 1.3.
When working at full power it produces a toroidal field
of 0.3 Tesla and a plasma current of 290 thousand Amps.
It has been found that the plasma stability can be a
factor of 10 or less than conventional Tokamaks yet
produce the same plasma current.
Some interesting results
are:-
- Confinement time is
significantly better than conventional
Tokamaks.
- World record energy efficiency
has been achieved when using the neutral beam
injection.
CONCLUSIONS:
It is clear that there are two
distinct approaches to producing a feasible nuclear
fusion reactor. Each one has been better and more
powerful then its predecessor. All the right conditions
are being met to produce nuclear fusion, however as long
as the budgets are being split, neither group can achieve
what they really intend to. What is needed is a definite
proposals by governing bodies to fund these projects to
get their full capability. The ITER approach is a very
good example for this, but in the last few weeks budget
cuts have hampered its development. It now has to be
cheaper than what was originally proposed. Staff have
lost their jobs and although they may find employment
elsewhere it takes out of the project brilliant minds
that helped develop it in the first place. Not a single
scientist believe that viable nuclear fusion will be
available within the next fifty years and if more cuts
are to take place it will be even longer. It is very
important I think that these programs be funded fully so
that in the future when fossil fuels are running low we
will have sufficient energy to fulfil our
needs.
Bibliography:
Alejaldre, C (1996)
Stellarators Physics World Oct pp
25-29
Conn, R.W (1983) The
Engineering Of Magnetic Fusion Reactors Scientific
American Oct Vol 249 (4) pp 44-55
Craxton, R.S McCrory, R.L Soures,J
(1986) Progress In Laser Fusion Scientific
American Aug Vol 255 (2) pp 60-71
Furth,H.P (1995) Fusion
Scientific American Sep Vol 273 (3) pp 140-142
Goddard,A (1998) Fusion
Partners Vote To Cut Costs Physics World Mar
p7
Nevins,B (1996) Magnetic
Turnaround For Fusion Plasmas Physics World Mar p
23
OBrien,M Robinson,D (1994)
Setting The Course For A Commercial Power
Plant Atom June/July (434) pp 32-35
Thomassen,K.I (1993) Progress
In Magnetic Fusion Energy Research Proc.IEEE Mar
Vol 81 (3) pp 390-398
RETURN
TO ARTICLES PAGE